IN LATE 1998, Douglas Jenson was dying. Diagnosed with chronic myelogenous leukemia (CML) only a few months earlier, the 65-year-old Oregon native was already out of options. The drug he was on, interferon, didn’t work and was making Jenson sicker. Younger patients could opt for a bone marrow transplant—a high dose of chemotherapy or radiation to kill the cancer cells, followed by an infusion of healthy blood stem cells. But because of his age, Jenson was told he had less than a 10 percent chance of surviving the transplant, even with a perfect marrow match. So when his doctor told him about a researcher who was starting a phase I trial of a new drug, called STI571, he didn’t take too much time deciding whether to participate. After all, doing anything was better than just lying there in his hospital bed, waiting to die.
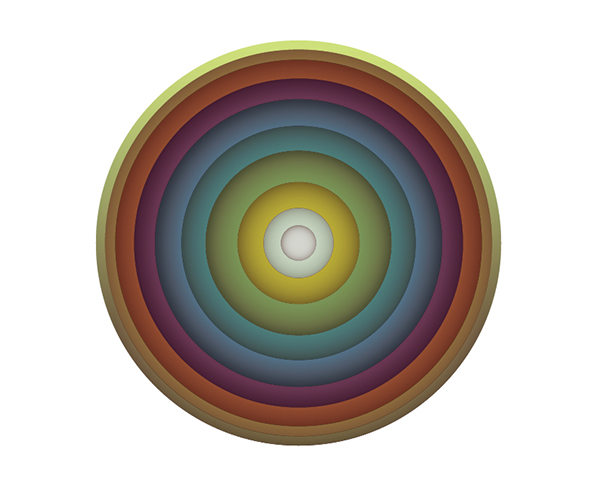
Within weeks, Jenson walked out of the hospital. Such miraculous recoveries are almost unheard of in the world of cancer treatments, especially in clinical trials. Then again, not every drug has the same backstory as STI571, now known as Gleevec (imatinib). “I had been told I had two or three years to live,” says Jenson, now 78. “One day I’m putting my affairs in order, the next I’m completely fine. That was more than 12 years ago. I take 400 milligrams of imatinib a day with breakfast. Actually, my knees bother me more than my leukemia.”
In 2005, Ryan Corbi’s experience was less dramatic but no less miraculous. Then a senior Villanova University near Philadelphia, Corbi went to see his doctor after he began experiencing stomach pain. Blood tests revealed his white blood cell count was sky-high. Within days, Corbi learned that, like Jenson, he too had CML. But unlike Jenson, Corbi was told he had a “good” cancer that could be treated with Gleevec. “I take a pill every day, and will probably have to for the rest of my life,” says Corbi. “To be honest, it’s not like having cancer or even a chronic disease, it’s like not having anything. I mean, I live a completely normal life.”
The New Standard of Care
In May of 2001, less than three years after the beginning of the first clinical study and in near record time, the U.S. Food and Drug Administration (FDA) approved Gleevec. It became the standard of care for CML almost the instant it appeared on the market.
Gleevec works by specifically targeting diseased cells. That’s why it doesn’t cause the often excruciating side effects that can occur with radiation and chemotherapy, which indiscriminately kill all dividing cells, both healthy and cancerous. More important, Gleevec was proof-of-concept that understanding and specifically targeting subtle molecular changes that turn cells cancerous can produce more effective, less toxic treatments, and it has reshaped the cancer research field.
“There are hundreds of molecularly targeted drugs in the pharmaceutical pipelines, and that number is growing,” says Brian Druker, the medical oncologist at Oregon Health and Science University (OHSU) in Portland who led the team that developed Gleevec. Already dozens of carefully designed targeted therapies—like Erbitux (cetuximab) for colon cancer, Sutent (sunitinib) for some kidney cancers, and Tykerb (lapatinib) for breast cancer—have joined Gleevec as standard treatments.
From the double helix to Gleevec.
- 1953: James Watson and Francis Crick famously elucidate the structure of the DNA double helix.
- 1956: Swedish geneticists decisively prove that humans have 46 chromosomes, not 48.
- 1959: Paris biologist Jerome Lejeune links Down syndrome to an extra copy of the 21st chromosome.
- 1960: Peter Nowell and David Hungerford report an abnormally small chromosome in cells taken from patients with chronic myelogenous leukemia (CML). The dwarfed version of chromosome 22 will later be dubbed the Philadelphia chromosome.
- 1960s: Extra or missing chromosomes are linked to a number of life-threatening birth defects and lesser-known genetic disorders like Turner syndrome and Klinefelter syndrome.
- 1972: Janet Rowley discovers the 9;22 translocation: a broken piece of chromosome 22 attached to chromosome 9.
- 1980s: Researchers investigate the biochemical pathway, BCR-ABL, that is created by the 9;22 translocation.
- Early 1990s: Brian Druker and his colleagues begin to search for a chemical compound that will target and disrupt the BCR-ABL pathway.
- Late 1990s: A phase I clinical trial of Gleevac (imatinib) gets underway; nearly all of the 54 CML patients enrolled in the trial respond to the drug.
- May 2001: Less than three years after the beginning of the first clinical study and in near record time, the U.S. Food and Drug Administration approves Gleevec.
A Serendipitous Discovery
All of these drugs, in some sense, originate from a serendipitous observation that the pathologist Peter Nowell made more than 50 years ago. Nowell had just taken a position at the University of Pennsylvania in Philadelphia after finishing up a two-year stint in the Navy, where he studied leukemia cells. “The university just let me do whatever I wanted to do,” says Nowell, “so I just carried on messing around with leukemia cells.” His research required that he wash the cells with tap water before staining them, which happened to make the cells swell up and the chromosomes easier to see. Nowell wasn’t a geneticist, but even he could see that there was an abnormally small chromosome present in some of the cells.
At the time, genetic studies were still crude— it was, after all, less than a decade since James Watson and Francis Crick famously elucidated the structure of the DNA double helix. And it was only a few years earlier, in 1956, that geneticists in Sweden had published a study that decisively proved that humans had 46 chromosomes (it had been assumed to be 48 since the 1920s). Once scientists knew what was normal, it was easier to identify what was not, making it possible to link chromosomal abnormalities to disease. In 1959, a Paris biologist linked Down syndrome to an extra copy of the 21st chromosome. By the early ’60s, extra or missing chromosomes were linked to other life-threatening birth defects and a constellation of lesser-known genetic disorders like Turner syndrome and Klinefelter syndrome.
Intrigued by what he had found, Nowell recruited a graduate student, David Hungerford, from Philadelphia’s Fox Chase Cancer Center and the pair began taking a closer look at blood samples taken from leukemia patients. The two quickly noticed that every single case of CML had the same abnormally tiny chromosome. When Nowell and Hungerford wrote up their discovery for publication in a 1960 issue of the journal Science, most of their colleagues regarded it as a curiosity. “I remember one of the reviewers telling me he thought it was interesting but that it had no scientific significance,” says Nowell. “They published it anyway, though.”
Nowell and Hungerford didn’t know it, but they had identified the first-ever genetic signature for a cancer. They did know, though, that their finding strongly hinted that the mutation was the cause, rather than the result, of the leukemia. “That was good news and bad news,” says Nowell. “The good news is that we could suspect what the problem was; the bad news is, we didn’t have the technology to do anything about it at the time.”
Before long, another team of researchers dubbed the tiny chromosome, a dwarfed version of chromosome 22, the Philadelphia chromosome.
An Intriguing Observation
Nowell and Hungerford soon turned their attention to other areas, and the chromosome remained a mystery until it piqued the curiosity of Janet Rowley, a researcher and physician who was working part-time while raising four sons. She had become intrigued by genetics when she had worked with Down syndrome children, and she continued to follow the research. In 1972, sitting at her dining room table, Rowley was looking at pictures of chromosomes from CML patients who had been treated by a former professor. She noticed something odd about the Philadelphia chromosome: It was broken. It was small because a piece of it was missing. Even odder, Rowley found that the missing piece was glued on to chromosome 9.
Rowley knew it was unlikely that finding a piece of chromosome 22 attached to chromosome 9 (called a 9;22 translocation) was simply a random by-product of the cancer. A random mutation wouldn’t be that consistently present in CML patients and absent in non-CML patients. Rowley believed she had found a smoking gun, and that it was the mutation that caused CML and not, as others had postulated, that the cancer had caused the mutation. She spent the next few years fervently arguing her case in scientific publications and at scientific meetings—but her colleagues paid little heed.
A decade later Rowley was vindicated, after geneticists showed that chromosome 22’s missing piece joined up with chromosome 9 in the neighborhood of a cancer-related gene. The new hybrid gene began making a protein that acts like a molecular “on” switch that causes cells to replicate out of control. This newly discovered biochemical pathway was later named BCR-ABL, after the particular genes—BCR and ABL—located at the breakpoints of the two chromosomes.
The 9;22 translocation is a glitch, an accidental mutation that occurs when the stem cells in the bone marrow divide. The mutation initially occurs in just one white cell, but each time that cell and its offspring divide, they pass on the translocation. The fused gene makes a product that tells cells to replicate continuously, causing leukemia.
It wasn’t good news, as Nowell would put it, but it was better news. Researchers now had a clear target to aim for: If they could interrupt the BCR-ABL pathway—the product of the translocation—the leukemia should shut down.
Newer drugs are available when Gleevec doesn’t work.
For most chronic myelogenous leukemia (CML) patients, Gleevec (imatinib) is nothing less than miraculous, wiping out the disease and restoring a normal life expectancy. But Gleevec doesn’t work for everyone—as many as 20 percent of patients have tumors that eventually stop responding to Gleevec in the first five or six years. Studies suggest that Gleevec works best in patients who are diagnosed in the early stages of the disease. Over time, mutations can develop that change the shape of the drug’s target, the BCR-ABL protein, so that Gleevec no longer fits in the slot. It’s “like sticking gum in a lock, so the key doesn’t work,” explains Charles Sawyers, a medical oncologist at Memorial Sloan-Kettering Cancer Center in New York City.
In 2006 and 2007, the U.S. Food and Drug Administration approved two new drugs, Sprycel (dasatinib) and Tasigna (nilotinib), as second-line therapies for CML patients who don’t respond to Gleevec. Like Gleevec, both work by targeting BCR-ABL, but they are able to bind to it more strongly or in a slightly different way. In 2010, the two drugs were also approved as first-line therapies after studies suggested that in most cases they are superior to Gleevec at inducing complete remissions.
These two newer drugs may continue to provide miraculous results for the vast majority of CML patients. But resistance to them could also become a problem. “The most significant mechanism of treatment failure is likely to be the T315I mutation, known as the gatekeeper, which confers resistance to all three drugs,” says Sawyers. This genetic abnormality may exist in newly diagnosed patients or develop later during treatment. Thankfully, a newer drug, ponatinib, has shown promising results in clinical trials of CML patients with the T315I mutation.
A Critical Collaboration
The lure of CML—a cancer caused in a clearly defined way, by a specific protein that’s produced consistently by a known genetic abnormality—was irresistible to Druker when he entered the research field. During medical school, he had become convinced that the best way to fight cancer was at the molecular level. “I wouldn’t exactly say I was obsessed with the idea,” says Druker. “But I couldn’t think of any reason why it wouldn’t work.” So in the early 1990s, intent on developing a targeted cancer therapy, he decided to take up the gauntlet.
As an oncologist at the Dana-Farber Cancer Institute in Boston in the late 1980s, Druker had seen his share of CML patients. And he knew firsthand how little there was to offer them. Druker may not have been able to think of any reason why targeting the BCR-ABL pathway wouldn’t work, but his superiors believed the idea wouldn’t amount to much. Undeterred, Druker moved across the country in 1993 and set up shop in Oregon.
He also began collaborating with Alex Matter and Nicholas Lydon, like-minded biochemists at the drug company that would eventually become Novartis. Lydon had become proficient in a high-tech method of pharmaceutical testing called “high-throughput screening” that enables thousands of chemical compounds to be quickly tested for potential activity against a particular biochemical pathway. Using this method, Lydon’s lab would find compounds that had potential activity against the BCR-ABL pathway and send them to Druker for testing. Eventually, they hit on a winner—a molecule that killed every leukemia cell in Druker’s petri dishes. But just when it looked like the researchers were on to something, Lydon’s company underwent a merger and Lydon left. Without Lydon’s advocacy, the executives weren’t convinced that Druker’s new drug was worth the substantial investment of clinical trials. After all, there wasn’t a lot of profit potential in a drug for a disease that’s diagnosed in only about 5,000 Americans a year.
Druker rallied colleagues at OHSU and other institutions for support. Ultimately, he found an advocate in Matter, who had stayed with the company and who went on to champion the drug through further trials. “At one point, I even thought about passing around a petition,” says Druker. “In 1996, I had a patient who was a dot-commer. … His leukemia was poorly controlled, and I remember at one point, he said, ‘Can’t you just go upstairs to the lab and get me some of that drug you’re working on?’ He later died. That sort of thing is really motivating. I’m not sure that if I had been just a researcher, I would have had the same level of commitment.
Druker’s dedication paid off. Phase I clinical trials are typically considered very successful if 10 to 20 percent of the participants show a significant positive response to the drug being tested. The first imatinib trial had almost a 100 percent response rate, with nearly all of the 54 patients seeing Lazarus-like responses like Jenson’s. “I remember thinking that it was almost too good to be true,” says Druker.
In fact, it was better. Less than three years after Jenson enrolled in Druker’s phase I trial, Gleevec was giving new hope to CML patients—as Corbi’s more encouraging experience with CML shows. And while, over time, about 20 percent of patients develop some resistance to Gleevec, Druker and others have found similar drugs that work when Gleevec no longer does. Moreover, Gleevec isn’t effective in only CML. It has been approved for gastrointestinal stromal tumors (GIST), certain skin cancers and other types of leukemia.
“What was really important about Gleevec was that it paved the way for other drugs to target molecular events in cancer,” says Jeff Boyd, the executive director of the Cancer Genome Institute at Fox Chase Cancer Center. “There are 30 targeted therapies now FDA-approved, and they all go back to Gleevec.”
That number is expected to grow as technological advances in high-throughput drug screening and cancer genome screening and analysis get cheaper and faster, says Charles Sawyers, a medical oncologist at Memorial Sloan-Kettering Cancer Center in New York City who helped develop Gleevec, and who has also helped develop a targeted therapy for prostate cancer called MDV3100 that is now in phase III clinical trials. “It’s only been in the past few years that we’ve really seen the technology take off—there are a lot of really promising drugs in the works.”
Corbi is fine with the idea of being on Gleevec for the rest of his life. But he doesn’t think he will have to be. “If you think about how far this whole process has come in 50 years and especially the last 10 years, I’m pretty sure that something that knocks it out completely will come along in my lifetime,” says Corbi. “I have a lot of faith in that.”
Cancer Today magazine is free to cancer patients, survivors and caregivers who live in the U.S. Subscribe here to receive four issues per year.