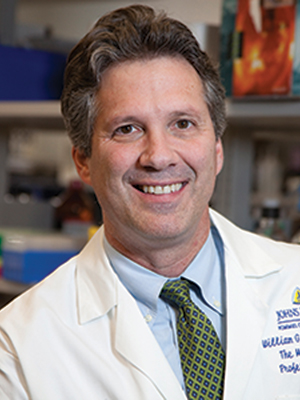
William G. Nelson, MD, PhD Photo by Joe Rubino
Many types of cancer are caused by gene mutations acquired during a person’s lifetime. Systematic cataloging of these acquired gene defects has led to improved screening, detection, diagnosis, prevention and treatment.
Next-generation genome sequencing, which quickly delivers an inventory of all defective genes in cancer cells or tissues, has streamlined drug development. As a result, new cancer treatments can be introduced and evaluated more rapidly and reliably, particularly molecularly targeted agents to treat cancers with specific gene defects. More efficient development of cancer treatments is paying off: The U.S. Food and Drug Administration approved 13 oncology drugs in 2013, 10 in 2014 and 17 in 2015.
Yet the sheer quantity of data generated about human cancers creates a daunting challenge. A report in the July 2015 PLOS Biology by Zachary D. Stephens and colleagues predicts that by 2025, as many as 100 million to 2 billion genome sequences, many from cancer, will take up between 2 and 40 exabytes of data storage (1 exabyte is 1018 or a quintillion bytes = 1,000,000,000,000,000,000 bytes). Cancer genome sequencing is becoming a significant driver of big-data science, but one worry is that the large number of acquired gene defects, between 1,000 and 10,000 for each cancer case, may undermine attempts to make sense of the consequences of each gene alteration for prognosis or treatment.
To make the best use of cancer genome sequencing, basic research into all 20,000 human genes is needed to better understand how they form normal cells and tissue and how dysfunctional genes create cancers. This discovery research is as vital for finding the ultimate cure for cancer as is translational and clinical research that is closer to benefiting patients.
The best discovery research is unfettered, driven by intellectual curiosity and conceptual advancement. As an example, research in the 1970s by Christiane Nüsslein-Volhard and Eric Wieschaus on how a fertilized fruit fly egg can give rise to a complicated segmented larva with a defined head and tail seemed a far cry from cancer research. Yet this seemingly unrelated avenue of research ultimately led to an effective cancer treatment.
Over the years following the research of Nüsslein-Volhard and Wieschaus, the desire to understand how body segments and appendages form during embryonic development intensified and sparked interest in a large number of researchers. Among them was Philip Beachy, who focused on a fruit fly gene called hedgehog, which if defective makes fly embryos look stubby and hairy (like a hedgehog). Beachy later found that when one of the three hedgehog genes in mammals, called sonic hedgehog, was faulty, mice exhibited marked defects, such as the presence of a single eye. Beachy and his research team realized this “cyclops” deformity was similar to defects seen in offspring of livestock that graze on corn lilies, attributed to a steroid-like corn-lily chemical called cyclopamine. Sure enough, cyclopamine was found to disrupt sonic hedgehog-associated processes.
Sonic hedgehog and cyclopamine might have remained of interest only to researchers studying embryonic development and birth defects until overactivity of hedgehog-associated signaling was found to be responsible for many cases of basal cell carcinoma of the skin and of medulloblastoma in the central nervous system. The intense hedgehog signaling in these cancers was a result of acquired defects in hedgehog-related genes like PTCH and SMO. Cyclopamine and related compounds inhibited the hedgehog signaling and stopped the cancer growth. In 2012 the FDA approved a hedgehog antagonist, Erivedge (vismodegib), to treat basal cell carcinoma.
Imagine if researchers like Beachy had not studied hedgehog signaling in fruit flies and mice before genome sequencing revealed frequent mutations in the hedgehog-related genes in basal cell cancers and medulloblastomas. There would have been no rationale to create a drug like Erivedge.
More such discovery research is needed. Yet many worry that discovery research—largely supported by the National Institutes of Health and the National Science Foundation—may become critically underfunded.
A more deliberate investment in discovery research could reveal more opportunities for developing cancer treatment by making better sense of the acquired gene defects in different cancers.
Cancer Today magazine is free to cancer patients, survivors and caregivers who live in the U.S. Subscribe here to receive four issues per year.