RANDY WATNICK’S PURSUIT of a better drug against metastatic cancer began late at night in early 2005. His infant daughter had woken up in tears at their home in Newton, Mass., near Boston. The molecular biologist climbed out of bed, scooped up the sobbing baby, and helped her get back to sleep. Normally able to maintain a healthy distance between work and family, Watnick was unable to fend off thoughts about tumor biology.
“I just couldn’t sleep,” he says. “My daughter woke me up, but what kept me awake was work.”
He couldn’t help it—work was frustrating. Barely a year earlier, Watnick had joined the Vascular Biology Program at Children’s Hospital Boston. His research centered on understanding how primary tumors use their environments to grow, the cancer biology equivalent of a botanist figuring out how an invasive weed thrives in a garden. Watnick was particularly interested in understanding the role of the soil, or stroma—healthy cells that provide fertile ground for cancer’s growth—but the work wasn’t going well.
His frustration wasn’t with the lab. It was a case of molecular mismatch. Watnick, 40, could obtain tumor samples, and he could obtain stromal cell samples, but not from the same patients. Tumors—even of the same type of cancer—can vary from person to person, and mismatched cells might not lead to reliable results. Plus, he felt intimidated by other players in the field. Established researchers with bigger labs and more funding were already deciphering the molecular conversations between diseased cells and healthy host cells. Watnick, whose lab was then barely older than his daughter, couldn’t imagine how his work would make a difference.
“I thought, ‘I can’t compete with them,’ ” he says. “That was a crowded space.”
Watnick slept a bit that night, and when he awoke the next morning, he knew what he would do: Study metastatic disease. Few researchers were studying the mechanics of metastasis, and even though his work wasn’t guaranteed to be useful, it could potentially play a role in the development of a therapy to help prevent cancer’s spread or to treat patients with stage IV disease.
“People die of metastatic disease,” he says. “They don’t generally die from primary tumors.”
The decision seemed momentous at the time, but Watnick was closer than he had imagined to a potential advance. In his earlier research on primary cancers, he had investigated how molecules produced by tumors corrupt nearby healthy cells to obtain the fuel they need to grow. That work had grounded him with the confidence to shift his perspective: He would look for proteins that could make healthy tissue inhospitable to metastases. This unconventional research project was by no means a sure thing—even the most encouraging laboratory studies only rarely result in human benefit—but Watnick wasn’t one to shy away. And, though he didn’t know it, he was already on his way to discovering a promising protein that might act as a metastatic roadblock.
The Anatomy of Metastasis
Metastasis begins when cancerous cells leave the primary tumor and circulate through the body, traveling in blood or lymph. Despite the best efforts of the human body’s patrolling immune cells, these seeds—called circulating tumor cells—can invade distant organs and grow out of control. Metastatic disease is diagnosed when cancerous cells from one organ are found in another organ, such as when a mass of breast cancer cells are discovered in the brain. In rare cases, doctors will find metastatic disease without knowing where the primary tumor started.
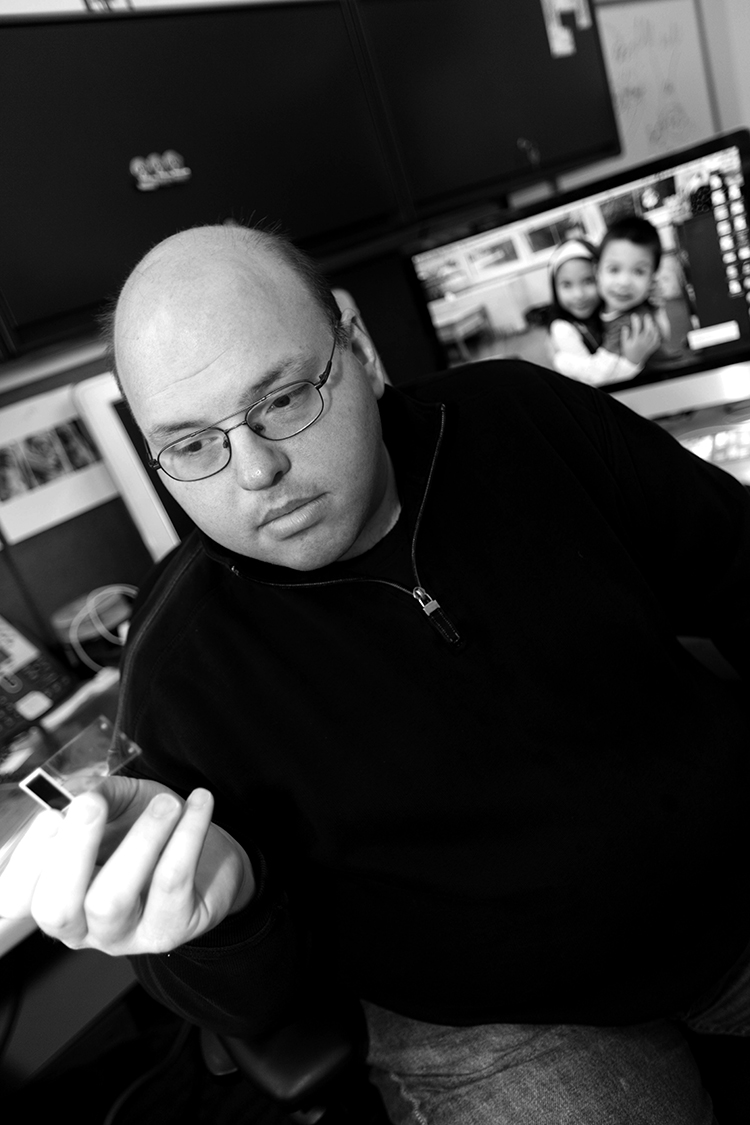
Searching for clues to the prevention or treatment of metastases, Randy Watnick has focused his research on cancers that don’t metastasize, or that metastasize slowly. Photo by Doug Sanford
About 30 percent of new cancer patients face an initial diagnosis of metastasis, and nine out of 10 cancer deaths are due to metastatic disease. But these broad statistics fail to show the radical diversity of metastatic disease among different kinds of cancer and among individual patients. Part of the complexity is that tumors contain a variety of diseased cells, which arise from different types of genetic mutations. So even when two people are diagnosed with the same type of cancer, they might have drastically dissimilar diseases.
Metastasis is often described as something that happens late in the disease, after tumors have had a chance to develop and shed cells into the body. As a tumor grows, its cells divide rapidly and undergo multiple mutations, accumulating changes and eventually releasing tumor cells. Experimental evidence linking large tumors to a greater likelihood of metastasis supports this hypothesis.
Other studies suggest the picture may be more complex: Metastatic cells at the outset may differ from cancer cells that will remain behind in primary tumors—mutating and evolving in different ways. If this is the case, treatment against one type of cancer cell in the primary tumor (the kind that will stay put regardless) may not work against other types (the ones that can develop the mutations necessary to spread). Both scenarios are unsettling because they suggest that cancer spreads before it becomes detectable. “When someone receives a diagnosis of cancer, that tumor has been there for months already,” says Joan Massagué, the director of the Metastasis Research Center at the Memorial Sloan-Kettering Cancer Center in New York City. “And that tumor has been fed by blood vessels, and all of those vessels are open windows for cancer cells to escape. The majority of the [escaped] tumor cells die, but some are going to manage to stay alive, hiding in the bone marrow, hiding in the lungs, in tissues that are large and have lots of capillary vessels.”
Scientists like Watnick and Massagué who study the complexity of metastatic disease toil in uncertainty. They may spend years hoping—but not knowing—if experiments will yield useful results. “You make a discovery, but not all discoveries provide the opportunity for the development of new drugs,” says Massagué. “Some do, some don’t.” Nevertheless, he’s optimistic that current research will yield treatments that extend patients’ lives. In the last 10 years, Massagué says, studies have begun to crack open the mysterious machinery of metastatic disease, and knowledge is accumulating quickly. But the silent nature of metastasis—hidden but growing—makes it a particularly daunting research subject.
Although research has led to new treatments, most metastatic disease remains incurable. For individuals with metastatic disease, when hope arrives, it arrives in “these very tiny increments,” says Suzanne Hebert, the vice president of the Metastatic Breast Cancer Network, an organization that strives to raise awareness of metastatic patients’ needs. In 2004, Hebert was diagnosed with stage IV breast cancer. She says she’s “guarded” when she hears news about new treatments for metastatic disease.
“After more than eight years of living with this, I’ve seen so many things that sound like the next great thing,” she says. “You never hear anything else.”
As an example, she points to the drug Halaven (eribulin), which was approved in November 2010 by the U.S. Food and Drug Administration (FDA) for use against metastatic breast cancer. News articles heralded the introduction of a “successful” new drug, but clinical studies showed that it extends life by an average of two and a half months. It was hardly the magic bullet for which patients like Hebert are waiting.
“That’s not really something to bring the trumpets out about, but that’s the best that we get,” she says. Still, it’s better than nothing. “I’m 46 and the mother of two,” says Hebert. “I’ll take it.”
Communities and support services that serve patients with metastatic cancer are growing.
Two years ago, while driving down the highway, Suzanne Hebert saw a billboard advertising breast cancer treatment at a nearby cancer center. It featured a woman and four words: I never gave up. Hebert wondered, “So the 40,000 women who died last year gave up?”
For Hebert, who has been living with metastatic breast cancer since 2004, the ad touched a nerve. In 2011, more than half a million Americans died of cancer, with about 90 percent of those deaths due to metastases. All cancers can metastasize, with the lungs and liver being the most common organs to which cancer spreads.
Hebert says that, like the billboard, the words commonly used to talk about cancer don’t always resonate with patients who have metastatic disease. For instance: “You think of a ‘survivor’ as someone who has gone through something like a plane crash, where the event is over,” she says. But for people with metastatic cancer, “there is no end point. We’re on treatment forever. We’re incurable, and our disease is not going to go away.”
Communities and support services that serve patients with metastatic cancer are growing. Case in point: Eight years ago, Jane Soyer and Nina Schulman launched the Metastatic Breast Cancer Network, for which Hebert is now the vice president. “Those of us with metastatic disease were the unheard voices in the cancer community,” Schulman wrote in 2007, a year before she died of breast cancer. Today, the organization has 2,000 members worldwide, helping them to act as their own advocates and raising awareness of the needs of metastatic breast cancer patients.
And breast cancer patients aren’t the only ones who can find help with their specific needs as they cope with metastatic cancer. Individuals with all types of metastatic disease can often find support from both local and national organizations. Here are a few places to look:
- CancerCare. This national nonprofit offers educational workshops and support groups—by telephone, in person and online—for people with metastatic disease.
- Major cancer centers. These large institutions frequently host support groups aimed at specific patients, including those with metastatic disease.
- Hospitals and local health centers. Social workers or health professionals often lead support groups at regional centers.
Fighting Cancer With Cancer
The Vascular Biology Program at Children’s Hospital Boston was established by Judah Folkman, a visionary cancer researcher who, at least for a while, seemed to have made headway in the quest for a magic bullet—the kind desperately needed by patients like Hebert.
In 2003, Folkman recruited Watnick from his position at the Whitehead Institute in nearby Cambridge, Mass. Decades earlier, in the 1970s, Folkman had championed the idea that a tumor could be starved by cutting off its blood supply. His work led to the development of a new class of cancer drugs called angiogenesis inhibitors. (Angiogenesis is the formation of blood vessels.) Avastin (bevacizumab) was the first angiogenesis inhibitor to extend patients’ lives, and over the last eight years, it has boasted FDA approval for the treatment of some types of colorectal cancer, lung cancer, kidney cancer, brain cancer, and, until last year, breast cancer. Following on Avastin’s heels, more than 10 additional angiogenesis inhibitors have been approved by the FDA, and at least 50 more are now in clinical trials.
Yet, so far, angiogenesis inhibitors have not lived up to their initial promise. Although some patients experience quick responses, most develop resistance to the drugs over time. And in November 2011, the FDA revoked its 2008 approval of the use of Avastin for metastatic breast cancer, citing poor performance in recent clinical trials and a high risk of serious side effects. Researchers continue to explore how current angiogenesis inhibitors or their pharmaceutical descendants might aid in efforts to cripple cancer’s growth.
By the time Folkman recruited Watnick, the younger scientist had established himself as a promising researcher in the angiogenesis field. And Watnick soon felt right at home. Folkman, who died in 2007, encouraged his team of researchers to take bold risks, says Watnick. Before he joined Folkman’s department, Watnick and his colleagues had demonstrated the angiogenesis-inhibiting behavior of a protein called thrombospondin-1, or Tsp-1, publishing their results in the scientific journal Cancer Cell in 2003. Folkman was impressed and recognized a kindred creative spirit of investigation.
“Dr. Folkman believed anything was possible,” Watnick says. “He had the spirit of trying new things and not being afraid to fail. When the director of your program gives you the freedom and encouragement to do new things, that gives you a lot more confidence to go out and do them.”
So in 2005, following that near-sleepless night, Watnick felt comfortable that he could choose an unorthodox research project. Other scientists had already discovered that when primary tumors start to metastasize, they release proteins that act as a first wave of invasion, preparing healthy tissue to support the seeds of a secondary growth. Naturally, some researchers thought that if they could block that first surge of proteins with a drug, they might be able to stop metastasis before it starts.
Watnick flipped the problem around, focusing instead on cancers that don’t metastasize, or that metastasize slowly. Watnick wondered: Do these cancers themselves produce proteins that block the development of metastases? “My thought was that some tumors actually inhibit their own metastasis,” he says. If such a hypothetical metastasis-blocking protein existed, and he could find it, then he could potentially fight cancer with cancer.
A “Metastatic Switch”
Watnick first identified 20 possible proteins produced by tumors, and then whittled his list to two. By early 2006, he had narrowed the search to a large protein called prosaposin, or Psap. In healthy cells, the Psap protein plays a role in normal cellular metabolism. But Watnick had found that Psap also influences the behavior of Tsp-1—the angiogenesis-inhibiting protein—and when Psap is produced by a tumor, metastases are much less likely to develop. That suggested to him that Psap poisons the soil—the tissue where the seeds of secondary tumors might take hold. Still, Watnick remained skeptical of Psap’s potential until, one afternoon, Folkman wandered by and Watnick showed him his recent work.
“He said, ‘You could have a drug right there,’ ” Watnick recalls. “He was a very excitable person in general, so to have him latch on to that result so quickly got everyone in the lab more motivated and excited.”
Watnick was ecstatic. He had a starting place and Folkman’s blessing, and Psap was ready for testing. He began, as investigators do, with petri dishes, mice, and stored human tumor samples. The studies were small but promising: His team found low Psap levels in metastatic prostate and breast cancer cells they grew in lab dishes, as well as in metastatic prostate cancer samples obtained from patients. Cells engineered to have high Psap levels didn’t metastasize. And in mice injected with tumor cells that produced Psap, the researchers found that the disease was less likely to spread than in mice injected with tumor cells without Psap.
Watnick and his colleagues bundled these promising initial results—showing how they found Psap, its effects on mice, and its presence in human patients with metastatic prostate cancer—and sent their work to major scientific journals.
In 2009, Watnick and his team published their findings in the Proceedings of the National Academy of Sciences. They wrote that Psap has the potential to flip a “metastatic switch” by activating Tsp-1, which turns off the growth of new tumor-feeding blood vessels in parts of the body where metastases might grow. Psap, they believed, was prohibiting construction of new metastatic tumors.
Since then, Watnick and his colleagues have collected patient data showing that individuals whose tumors have high levels of Psap live longer than patients with low levels of the protein, and they are much less likely to develop metastases. In lab dishes and mouse studies, the researchers have seen Psap work against the most common types of metastases—in lung, liver and bone.
“We’re finding very striking results,” Watnick says. “When we treat with Psap [in mouse studies], we end up with tumors that are significantly smaller than in the control group.”
Watnick has submitted his most recent data for publication. He has also been meeting with potential investors, who—if Psap continues to demonstrate effectiveness at blocking metastasis in laboratory studies—may eventually finance the costly development and testing of a potential Psap-based drug.
Despite Psap’s promising start, however, Watnick cautions that the first clinical trials of any such drug in people are at least two years away. It’s also possible that his passionate investment could turn out to be a false start: Researchers have found thousands of molecules connected in some way to the spread of cancer. Most of these molecules never turn into plausible targets for cancer treatment—though a select few continue to advance slowly toward becoming potential new therapies.
If follow-up studies do continue to suggest that the protein can put up a metastatic roadblock, a Psap-based treatment would represent a new approach against cancer’s spread: It would work by boosting levels of a protective protein that’s already present in the human body, rather than introducing a chemical intended to attack individual cancer cells. What’s more, rather than trying to kill the seeds of metastasis, Psap would poison the soil where new tumors might try to grow, making those tissues poor environments for cancer’s growth. It wouldn’t be a cure, but the concept suggests the possibility of prolonging survival and turning some cancers into a chronic disease.
“This would probably be a treatment you have to take for your whole life,” Watnick says, looking forward. “But I would take a pill my whole life so I wouldn’t die from cancer.”
Cancer Today magazine is free to cancer patients, survivors and caregivers who live in the U.S. Subscribe here to receive four issues per year.